![]() | |
![]() | |
Names | |
---|---|
Other names Poliglusam; Deacetylchitin; Poly-(D)glucosamine; BC; Chitopearl; Chitopharm; Flonac; Kytex | |
Identifiers | |
CAS Number | |
3D model (JSmol) | |
ChemSpider | |
ECHA InfoCard | 100.122.259 ![]() |
UNII | |
CompTox Dashboard (EPA) | |
InChI
| |
SMILES
| |
Related compounds | |
Related compounds | D-glucosamine and N-acetylglucosamine (monomers) |
Except where otherwise noted, data are given for materials in their standard state (at 25 °C , 100 kPa).
![]() |
Chitosan /ˈkaɪtəsæn/ is a linear polysaccharide composed of randomly distributed β-(1→4)-linked D-glucosamine (deacetylated unit) and N-acetyl-D-glucosamine (acetylated unit). It is made by treating the chitin shells of shrimp and other crustaceans with an alkaline substance, such as sodium hydroxide.
Chitosan has a number of commercial and possible biomedical uses. It can be used in agriculture as a seed treatment and biopesticide, helping plants to fight off fungal infections. In winemaking, it can be used as a fining agent, also helping to prevent spoilage. In industry, it can be used in a self-healing polyurethane paint coating. In medicine, it is useful in bandages to reduce bleeding and as an antibacterial agent; it can also be used to help deliver drugs through the skin.
History
In 1799, British chemist Charles Hatchett experimented with decalcifying the shells of various crustaceans, finding that a soft, yellow and cartilage-like substance was left behind that we now know to be chitin. In 1859, French physiologist Charles Marie Benjamin Rouget found that boiling chitin in potassium hydroxide solution could deacetylate it to produce a substance that was soluble in dilute organic acids, that he called chitine modifiée. In 1894, German chemist Felix Hoppe-Seyler named the substance chitosan. From 1894 to 1930 there was a period of debate and confusion over the exact composition of chitin and particularly whether animal and fungal forms where the same chemicals. In 1930 the first chitosan films and fibres were patented but competition from petroleum-derived polymers limited their uptake. It was not until the 1970s that there was renewed interest in the compound, spurred partly by laws that prevented the dumping of untreated shellfish waste.
Manufacture


Chitosan is produced commercially by deacetylation of chitin, which is the structural element in the exoskeleton of crustaceans (such as crabs and shrimp) and cell walls of fungi. A common method for obtaining chitosan is the deacetylation of chitin using sodium hydroxide in excess as a reagent and water as a solvent. The reaction follows first-order kinetics though it occurs in two steps; the activation energy barrier for the first stage is estimated at 48.8 kJ·mol at 25–120 °C (77–248 °F) and is higher than the barrier to the second stage.
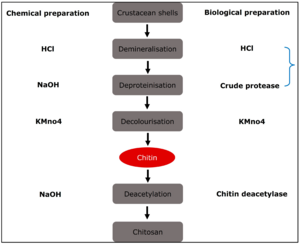
The degree of deacetylation (%) can be determined by NMR spectroscopy and the degree of deacetylation in commercially available chitosan ranges from 60 to 100%. On average, the molecular weight of commercially produced chitosan is 3800–20,000 daltons.
Nanofibrils have been made using chitin and chitosan.
Chitosan Chemical Modifications
Chitosan contains the following three functional groups: C2-NH2, C3-OH, and C6-OH. C3-OH has a large spatial site resistance and therefore is relatively difficult to modify. C2-NH2 is highly reactive for fine modifications and is the most common modifying group in chitosan. In chitosan, although amino groups are more prone to nucleophilic reactions than hydroxyl groups, both can react non-selectively with electrophilic reagents such as acids, chlorides, and haloalkanes to functionalize them. Since chitosan contains a variety of functional groups, it can be functionalized in different ways such as phosphorylation, thiolation, and quaternization to adapt it to specific purposes.
Phosphorylated Chitosan
Water-soluble phosphorylated chitosan can be obtained by the reaction of phosphorus pentoxide and chitosan under low-temperature conditions using methane sulfonic acid as the catalyst; phosphorylated chitosan with good antibacterial activity and ionic properties can be prepared by graft copolymerization of chitosan monophosphate.
The good water solubility and metal chelating properties of phosphorylated chitosan and its derivatives make them widely used in tissue engineering, drug delivery carriers, tissue regeneration, and the food industry.
In tissue engineering, phosphorylated chitosan exhibits improved swelling and ionic conductivity. Although its crystallinity is reduced, its tensile strength remains largely unchanged. These properties make it useful for creating scaffolds that can support bone tissue regeneration by binding growth factors and promoting stem cell differentiation into bone-forming cells. Additionally, to enhance the solubility of chitosan-based hydrogels at neutral or alkaline pH, the derivative N-methylene phosphonic acid chitosan (NMPC-GLU) has been developed. This material maintains good mechanical strength and improve cell proliferation, making it valuable for biomedical applications.
Thiolated Chitosan
Thiolated chitosan is produced by attaching thiol groups to the amino groups of chitosan using a thiol-containing coupling agent. The primary site for this modification is the amino group at the 2nd position of chitosan's glucosamine units. During this process, thioglycolic acid and cysteine mediate the reaction, forming an amide bond between the thiol group and chitosan. At a pH below 5, thiol activity is reduced, which limits disulfide bond formation.
The modified chitosan exhibits improved adhesive properties and stability due to the covalent attachment of the thiol groups. Lower pH reduces oxidation, enhancing its adhesion properties. Additionally, thiolated chitosan can interact with cell membrane receptors, improving membrane permeability and showing potential for applications in bacterial adhesion prevention, for example for coating stainless steel.
Ionic Chitosan
There are two main methods of chitosan quaternization: direct quaternization and indirect quaternization.
- The direct quaternization of chitosan amino acids treats chitosan with haloalkanes under alkaline conditions. Another method is the reaction of chitosan with aldehydes first, followed by reduction, and finally with haloalkanes to obtain quaternized chitosan.
- The indirect quaternization method refers to introducing small molecules containing quaternary ammonium groups into chitosan, such as glycidyl trimethyl ammonium chloride, (5-bromopentyl) trimethyl ammonium bromide, etc. Quaternary ammonium groups can further be introduced into the chitosan backbone via azide-alkyne cycloaddition, or by dissolving chitosan in alkali and urea and then reacting it with 3-chloro-2-hydroxypropyl trimethylammonium chloride, which provides a simple and green solution to achieve chitosan functionalization.
Cationic derivatives of chitosan have important roles in bioadhesion, absorption enhancement, anti-inflammatory, antibacterial and anti-tumor applications. Chitosan modified with quaternary ammonium groups is one of the most common cationic chitosan derivatives. Quaternized chitosan with a permanent positive charge has increased antimicrobial activity and solubility compared to normal chitosan.
Properties
The amino group in chitosan has a pKb value of ~6.5, which leads to significant protonation in neutral solution, increasing with increased acidity (decreased pH) and the %DA-value. This makes chitosan water-soluble and a bioadhesive which readily binds to negatively charged surfaces such as mucosal membranes. Also, chitosan can effectively bind to other surface via hydrophobic interaction and/or cation-π interaction (chitosan as a cation source) in aqueous solution. The free amine groups on chitosan chains can make crosslinked polymeric networks with dicarboxylic acids to improve chitosan's mechanical properties. Chitosan enhances the transport of polar drugs across epithelial surfaces, and is biocompatible and biodegradable. However, it is not approved by the FDA for drug delivery. Purified quantities of chitosan are available for biomedical applications.
Physicochemical Properties
Chitosan has excellent biological properties such as biodegradability, biocompatibility, antibacterial, anti-tumor, hemostatic and antioxidant properties. The biological properties of chitosan are closely related to its physicochemical structure, which includes the degree of deacetylation, water content, and molecular weight. Deacetylation refers to the process of removing the acetyl group from chitosan, and this process determines the content of free amine groups in chitosan. Studies have shown that chitosan has good solubility only when the degree of deacetylation is above 85%. The enhanced chitosan uptake is mainly due to the interaction of positively charged chitosan with cell membranes, activation of chlorine–bicarbonate exchange channels, and reorganization of proteins associated with epithelial tight junctions, thus opening epithelial tight junctions. Chitosan inhibits the growth of different bacteria and fungi, and the mechanism is influenced by several factors, including the degree of deacetylation, pH, divalent cations, and solvent type. Oligomeric chitosan penetrates cell membranes more easily than high molecular weight chitosan and inhibits bacterial growth by inhibiting RNA transcription.
Uses
See also: Chitosan nanoparticlesAgricultural and horticultural use
The agricultural and horticultural uses for chitosan, primarily for plant defense and yield increase, are based on how this glucosamine polymer influences the biochemistry and molecular biology of the plant cell. The cellular targets are the plasma membrane and nuclear chromatin. Subsequent changes occur in cell membranes, chromatin, DNA, calcium, MAP kinase, oxidative burst, reactive oxygen species, callose pathogenesis-related (PR) genes, and phytoalexins.
Chitosan was first registered as an active ingredient (licensed for sale) in 1986.
Natural biocontrol and elicitor
In agriculture, chitosan is typically used as a natural seed treatment and plant growth enhancer, and as an ecologically friendly biopesticide substance that boosts the innate ability of plants to defend themselves against fungal infections.
Degraded molecules of chitin/chitosan exist in soil and water. Chitosan applications for plants and crops are regulated in the USA by the EPA, and the USDA National Organic Program regulates its use on organic certified farms and crops. EPA-approved, biodegradable chitosan products are allowed for use outdoors and indoors on plants and crops grown commercially and by consumers.
In the European Union and United Kingdom, chitosan is registered as a "basic substance" for use as a biological fungicide and bactericide on a wide range of crops.
The natural biocontrol ability of chitosan should not be confused with the effects of fertilizers or pesticides upon plants or the environment. Chitosan active biopesticides represent a new tier of cost-effective biological control of crops for agriculture and horticulture. The biocontrol mode of action of chitosan elicits natural innate defense responses within plant to resist insects, pathogens, and soil-borne diseases when applied to foliage or the soil. Chitosan increases photosynthesis, promotes and enhances plant growth, stimulates nutrient uptake, increases germination and sprouting, and boosts plant vigor. When used as a seed treatment or seed coating on cotton, corn, seed potatoes, soybeans, sugar beets, tomatoes, wheat, and many other seeds, it elicits an innate immunity response in developing roots which destroys parasitic cyst nematodes without harming beneficial nematodes and organisms.
Agricultural applications of chitosan can reduce environmental stress due to drought and soil deficiencies, strengthen seed vitality, improve stand quality, increase yields, and reduce fruit decay of vegetables, fruits and citrus crops . Horticultural application of chitosan increases blooms and extends the life of cut flowers and Christmas trees. The US Forest Service has conducted research on chitosan to control pathogens in pine trees and increase resin pitch outflow which resists pine beetle infestation.

Chitosan has a rich history of being researched for applications in agriculture and horticulture dating back to the 1980s. By 1989, chitosan salt solutions were applied to crops for improved freeze protection or to crop seed for seed priming. Shortly thereafter, chitosan salt received the first ever biopesticide label from the EPA, then followed by other intellectual property applications.
Chitosan has been used to protect plants in space, as well, exemplified by NASA's experiment to protect adzuki beans grown aboard the space shuttle and Mir space station in 1997 (see photo left). NASA results revealed chitosan induces increased growth (biomass) and pathogen resistance due to elevated levels of β-(1→3)-glucanase enzymes within plant cells. NASA confirmed chitosan elicits the same effect in plants on earth.
In 2008, the EPA approved natural broad-spectrum elicitor status for an ultralow molecular active ingredient of 0.25% chitosan. A natural chitosan elicitor solution for agriculture and horticultural uses was granted an amended label for foliar and irrigation applications by the EPA in 2009. Given its low potential for toxicity and abundance in the natural environment, chitosan does not harm people, pets, wildlife, or the environment when used according to label directions. Chitosan blends do not work against bark beetles when put on a tree's leaves or in its soil.
Filtration
Chitosan can be used in hydrology as a part of a filtration process. Chitosan causes the fine sediment particles to bind together, and is subsequently removed with the sediment during sand filtration. It also removes heavy minerals, dyes, and oils from the water. As an additive in water filtration, chitosan combined with sand filtration removes up to 99% of turbidity. Chitosan is among the biological adsorbents used for heavy metals removal without negative environmental impacts.
In combination with bentonite, gelatin, silica gel, isinglass, or other fining agents, it is used to clarify wine, mead, and beer. Added late in the brewing process, chitosan improves flocculation, and removes yeast cells, fruit particles, and other detritus that cause hazy wine.
Winemaking and fungal source chitosan
Chitosan has a long history for use as a fining agent in winemaking. Fungal source chitosan has shown an increase in settling activity, reduction of oxidized polyphenolics in juice and wine, chelation and removal of copper (post-racking) and control of the spoilage yeast Brettanomyces. These products and uses are approved for European use by the EU and OIV standards.
Wound management
Chitosan-based wound dressings have been widely explored for a variety of acute and chronic wounds. Chitosan has the ability to adhere to fibrinogen, which produces increased platelet adhesion, causing clotting of blood and hemostasis. Chitosan hemostatic agents are salts made from mixing chitosan with an organic acid (such as succinic or lactic acid). Chitosan may have other properties conducive to wound healing, including antibacterial and antifungal activity, which remain under preliminary research.
Chitosan is used within some wound dressings to decrease bleeding. Upon contact with blood, the bandage becomes sticky, effectively sealing the laceration. Chitosan hydrogel-based wound dressings have also been found useful as burn dressings, and for the treatment of chronic diabetic wounds and hydrofluoric acid burns.
Chitosan-containing wound dressings received approval for medical use in the United States in 2003.
Temperature-sensitive hydrogels
Chitosan is dissolved in dilute organic acid solutions but is insoluble in high concentrations of hydrogen ions at pH 6.5 and is precipitated as a gel-like compound. Chitosan is positively charged by amine groups, making it suitable for binding to negatively charged molecules. However, it has disadvantages such as low mechanical strength and low-temperature response rate; it must be combined with other gelling agents to improve its properties. Using glycerolphosphate salts (possessing a single anionic head) without chemical modification or cross-linking, the pH-dependent gelation properties can be converted to temperature-sensitive gelation properties. In the year 2000, Chenite was the first to design the temperature-sensitive chitosan hydrogels drug delivery system using chitosan and β-glycerol phosphate. This new system can remain in the liquid state at room temperature, while becoming gel with increasing temperature above the physiological temperature (37 °C). Phosphate salts cause a particular behaviour in chitosan solutions, thereby allowing these solutions to remain soluble in the physiological pH range (pH 7), and they will be gel only at body temperature. When the liquid solution of chitosan-glycerol phosphate, containing the drug, enters the body through a syringe injection, it becomes a water-insoluble gel at 37 °C. The entrapped drug particles between the hydrogel chains will be gradually released.
Research
Chitosan and derivatives have been explored in the development of nanomaterials, bioadhesives, wound dressing materials, improved drug delivery systems, enteric coatings, and in medical devices.
Bioprinting

Bioinspired materials, a manufacturing concept inspired by natural nacre, shrimp carapace, or insect cuticles, has led to development of bioprinting methods to manufacture large scale consumer objects using chitosan. This method is based on replicating the molecular arrangement of chitosan from natural materials into fabrication methods, such as injection molding or mold casting. Once discarded, chitosan-constructed objects are biodegradable and non-toxic. The method is used to engineer and bioprint human organs or tissues.
Pigmented chitosan objects can be recycled, with the option of reintroducing or discarding the dye at each recycling step, enabling reuse of the polymer independently of colorants. Unlike other plant-based bioplastics (e.g. cellulose, starch), the main natural sources of chitosan come from marine environments and do not compete for land or other human resources.
3D bioprinting of tissue engineering scaffolds for creating artificial tissues and organs is another application where chitosan has gained popularity. Chitosan has high biocompatibility, biodegradability, and antimicrobial, hemostatic, wound healing and immunomodulatory activities which make it suitable for making artificial tissues.
Weight loss
Chitosan is marketed in a tablet form as a "fat binder". Although the effect of chitosan on lowering cholesterol and body weight has been evaluated, the effect appears to have no or low clinical importance. Reviews from 2016 and 2008 found there was no significant effect, and no justification for overweight people to use chitosan supplements. In 2015, the U.S. Food and Drug Administration issued a public advisory about supplement retailers who made exaggerated claims concerning the supposed weight loss benefit of various products.
Biodegradable antimicrobial food packaging
Microbial contamination of food products accelerates the deterioration process and increases the risk of foodborne illness caused by potentially life-threatening pathogens. Ordinarily, food contamination originates superficially, requiring surface treatment and packaging as crucial factors to assure food quality and safety. Biodegradable chitosan films have potential for preserving various food products, retaining their firmness and restricting weight loss due to dehydration. In addition, composite biodegradable films containing chitosan and antimicrobial agents are in development as safe alternatives to preserve food products.
Battery electrolyte
Chitosan is being investigated as an electrolyte for rechargeable batteries with good performance and low environmental impact due to rapid biodegradability, leaving recycleable zinc. The electrolyte has excellent physical stability up to 50 °C, electrochemical stability up to 2 V with zinc electrodes, and accommodates redox reactions involved in the Zn-MnO2 alkaline system. As of 2022 results were promising, but the battery needed testing on a larger scale and under actual use conditions.
References
This article incorporates text by Meng Zhang, Fengshi Zhang, Ci Li, Heng An, Teng Wan and Peixun Zhang available under the CC BY 4.0 license.
- Crini, Grégorio (December 2019). "Historical review on chitin and chitosan biopolymers". Environmental Chemistry Letters. 17 (4): 1623–1643. doi:10.1007/s10311-019-00901-0. ISSN 1610-3653.
- Shahidi, Fereidoon; Synowiecki, Jozef (1991). "Isolation and characterization of nutrients and value-added products from snow crab (Chionoecetes opilio) and shrimp (Pandalus borealis) processing discards". Journal of Agricultural and Food Chemistry. 39 (8): 1527–32. doi:10.1021/jf00008a032.
- ^ Sanjanwala, Dhruv; Londhe, Vaishali; Trivedi, Rashmi; Bonde, Smita; Sawarkar, Sujata; Kale, Vinita; Patravale, Vandana (28 November 2022). "Polysaccharide-based hydrogels for drug delivery and wound management: A review". Expert Opinion on Drug Delivery. 19 (12): 1664–1695. doi:10.1080/17425247.2022.2152791. ISSN 1742-5247. PMID 36440488. S2CID 254041961.
- ^ Sanjanwala, Dhruv; Londhe, Vaishali; Trivedi, Rashmi; Bonde, Smita; Sawarkar, Sujata; Kale, Vinita; Patravale, Vandana (1 January 2024). "Polysaccharide-based hydrogels for medical devices, implants and tissue engineering: A review". International Journal of Biological Macromolecules. 256: 128488. doi:10.1016/j.ijbiomac.2023.128488. ISSN 0141-8130. S2CID 265569145.
- Kou, Shijie (Gabriel); Peters, Linda M.; Mucalo, Michael R. (1 February 2021). "Chitosan: A review of sources and preparation methods". International Journal of Biological Macromolecules. 169: 85–94. doi:10.1016/j.ijbiomac.2020.12.005. hdl:10289/14259. ISSN 0141-8130. PMID 33279563. S2CID 227522332.
- Novikov, Vitaly Yu.; Derkach, Svetlana R.; Konovalova, Irina N.; et al. (30 March 2023). "Mechanism of Heterogeneous Alkaline Deacetylation of Chitin: A Review". Polymers. 15 (7): 1729. doi:10.3390/polym15071729. ISSN 2073-4360. PMC 10097213. PMID 37050343.
- Narudin, Nur Alimatul Hakimah; Rosman, Nurul ‘Aqilah; Shahrin, Ensan Waatriah ES; et al. (January 2022). "Extraction, characterization, and kinetics of N-deacetylation of chitin obtained from mud crab shells". Polymers and Polymer Composites. 30: 096739112211096. doi:10.1177/09673911221109611. ISSN 0967-3911.
- Mohammed, Musarrat H.; Williams, Peter A.; Tverezovskaya, Olga (1 June 2013). "Extraction of chitin from prawn shells and conversion to low molecular mass chitosan". Food Hydrocolloids. 31 (2): 166–171. doi:10.1016/j.foodhyd.2012.10.021. ISSN 0268-005X.
- de Alvarenga, Elson Santiago; Pereira de Oliveira, Cristiane; Roberto Bellato, Carlos (16 May 2010). "An approach to understanding the deacetylation degree of chitosan". Carbohydrate Polymers. 80 (4): 1155–1160. doi:10.1016/j.carbpol.2010.01.037. ISSN 0144-8617.
- Mima, Seiichi; Miya, Masaru; Iwamoto, Reikichi; Yoshikawa, Susumu (June 1983). "Highly deacetylated chitosan and its properties". Journal of Applied Polymer Science. 28 (6): 1909–1917. doi:10.1002/app.1983.070280607. ISSN 0021-8995.
- Jeffryes, C; Agathos, SN; Rorrer, G (June 2015). "Biogenic nanomaterials from photosynthetic microorganisms". Current Opinion in Biotechnology. 33: 23–31. doi:10.1016/j.copbio.2014.10.005. PMID 25445544.
- Qin, Y.; Li, P.; Guo, Z. Cationic chitosan derivatives as potential antifungals: A review of structural optimization and applications. Carbohydr. Polym. 2020, 236, 116002.
- Sahariah, P.; Masson, M. Antimicrobial chitosan and chitosan derivatives: A review of the structure–activity relationship. Biomacromolecules 2017, 18, 3846–3868.
- Jayakumar, R.; Selvamurugan, N.; Nair, S.k.V.; Tokura, S.; Tamura, H. Preparative methods of phosphorylated chitin and chitosan—An overview. Int. J. Biol. Macromol. 2008, 43, 221–225.
- Ardean, C.; Davidescu, C.M.; Nemeş, N.S.; Negrea, A.; Ciopec, M.; Duteanu, N.; Negrea, P.; Duda-Seiman, D.; Musta, V. Factors influencing the antibacterial activity of chitosan and chitosan modified by functionalization. Int. J. Mol. Sci. 2021, 22, 7449.
- Liu, L.; Miao, Y.; Shi, X.; Gao, H.; Wang, Y. Phosphorylated Chitosan Hydrogels Inducing Osteogenic Differentiation of Osteoblasts via JNK and p38 Signaling Pathways. ACS Biomater. Sci. Eng. 2020, 6, 1500–1509.
- Wei, J.; Xue, W.; Yu, X.; Qiu, X.; Liu, Z. pH Sensitive phosphorylated chitosan hydrogel as vaccine delivery system for intramuscular immunization. J. Biomater. Appl. 2017, 31, 1358–1369.
- Han, G.; Liu, S.; Pan, Z.; Lin, Y.; Ding, S.; Li, L.; Luo, B.; Jiao, Y.; Zhou, C. Sulfonated chitosan and phosphorylated chitosan coated polylactide membrane by polydopamine-assisting for the growth and osteogenic differentiation of MC3T3-E1s. Carbohydr. Polym. 2020, 229, 115517.
- Muzzarelli, R.A. Chitosan composites with inorganics, morphogenetic proteins and stem cells, for bone regeneration. Carbohydr. Polym. 2011, 83, 1433–1445.
- LogithKumar, R.; KeshavNarayan, A.; Dhivya, S.; Chawla, A.; Saravanan, S.; Selvamurugan, N. A review of chitosan and its derivatives in bone tissue engineering. Carbohydr. Polym. 2016, 151, 172–188.
- Bernkop-Schnürch, A.; Hornof, M.; Guggi, D. Thiolated chitosans. Eur. J. Pharm. Biopharm. 2004, 57, 9–17.
- Liu, X.; Li, X.; Zhang, R.; Wang, L.; Feng, Q. A novel dual microsphere based on water-soluble thiolated chitosan/mesoporous calcium carbonate for controlled dual drug delivery. Mater. Lett. 2021, 285, 129142.
- Khan, Ajahar; Alamry, Khalid A. (June 2021). "Recent advances of emerging green chitosan-based biomaterials with potential biomedical applications: A review". Carbohydrate Research. 506: 108368. doi:10.1016/j.carres.2021.108368.
- Mueller, C.; Verroken, A.; Iqbal, J.; Bernkop-Schnuerch, A. Thiolated chitosans: In vitro comparison of mucoadhesive properties. J. Appl. Polym. Sci. 2012, 124, 5046–5055.
- Laffleur, F. Evaluation of chemical modified hydrogel formulation for topical suitability. Int. J. Biol. Macromol. 2017, 105, 1310–1314.
- Federer, C.; Kurpiers, M.; Bernkop-Schnurch, A. Thiolated chitosans: A multi-talented class of polymers for various applications. Biomacromolecules 2020, 22, 24–56.
- Zhang, Y.; Zhou, S.; Deng, F.; Chen, X.; Wang, X.; Wang, Y.; Zhang, H.; Dai, W.; He, B.; Zhang, Q. The function and mechanism of preactivated thiomers in triggering epithelial tight junctions opening. Eur. J. Pharm. Biopharm. 2018, 133, 188–199.
- Mirani, Z.A.; Fatima, A.; Urooj, S.; Aziz, M.; Khan, M.N.; Abbas, T. Relationship of cell surface hydrophobicity with biofilm formation and growth rate: A study on Pseudomonas aeruginosa, Staphylococcus aureus, and Escherichia coli. Iran. J. Basic Med. Sci. 2018, 21, 760.
- Xu, L.Q.; Pranantyo, D.; Neoh, K.-G.; Kang, E.-T.; Fu, G.D. Thiol reactive maleimido-containing tannic acid for the bioinspired surface anchoring and post-functionalization of antifouling coatings. ACS Sustain. Chem. Eng. 2016, 4, 4264–4272.
- Wei, L.; Tan, W.; Wang, G.; Li, Q.; Dong, F.; Guo, Z. The antioxidant and antifungal activity of chitosan derivatives bearing Schiff bases and quaternary ammonium salts. Carbohydr. Polym. 2019, 226, 115256.
- Liu, W.; Qin, Y.; Liu, S.; Xing, R.; Yu, H.; Chen, X.; Li, K.; Li, P. Synthesis, characterization and antifungal efficacy of chitosan derivatives with triple quaternary ammonium groups. Int. J. Biol. Macromol. 2018, 114, 942–949.
- Shagdarova, B.; Lunkov, A.; Il′ina, A.; Varlamov, V. Investigation of the properties of N- chloride chitosan derivatives. Int. J. Biol. Macromol. 2019, 124, 994–1001.
- De Oliveira Pedro, R.; Schmitt, C.C.; Neumann, M.G. Syntheses and characterization of amphiphilic quaternary ammonium chitosan derivatives. Carbohydr. Polym. 2016, 147, 97–103.
- Tan, W.; Zhang, J.; Mi, Y.; Dong, F.; Li, Q.; Guo, Z. Synthesis, characterization, and evaluation of antifungal and antioxidant properties of cationic chitosan derivative via azide-alkyne click reaction. Int. J. Biol. Macromol. 2018, 120, 318–324.
- Song, H.; Wu, H.; Li, S.; Tian, H.; Li, Y.; Wang, J. Homogeneous synthesis of cationic chitosan via new avenue. Molecules 2018, 23, 1921.
- Mi, Y.; Tan, W.; Zhang, J.; Wei, L.; Chen, Y.; Li, Q.; Dong, F.; Guo, Z. Synthesis, characterization, and antifungal property of hydroxypropyltrimethyl ammonium chitosan halogenated acetates. Mar. Drugs 2018, 16, 315.
- Dong Woog Lee; et al. (2013). "Strong adhesion and cohesion of chitosan in aqueous solutions". Langmuir. 29 (46): 14222–14229. doi:10.1021/la403124u. PMC 3888206. PMID 24138057.
- Chanoong Lim; Dong Woog Lee; et al. (2015). "Contact time- and pH-dependent adhesion and cohesion of low molecular weight chitosan coated surfaces". Carbohydrate Polymers. 117 (6): 887–894. doi:10.1016/j.carbpol.2014.10.033. PMID 25498713.
- Lim, Chanoong; Hwang, Dong Soo; Lee, Dong Woog (1 May 2021). "Intermolecular interactions of chitosan: Degree of acetylation and molecular weight". Carbohydrate Polymers. 259: 117782. doi:10.1016/j.carbpol.2021.117782. ISSN 0144-8617. PMID 33674019. S2CID 232131640.
- Choi, Jieun; Hwang, Dong Soo; Lim, Chanoong; Lee, Dong Woog (15 January 2024). "Interaction mechanism between low molecular weight chitosan nanofilm and functionalized surfaces in aqueous solutions". Carbohydrate Polymers. 324: 121504. doi:10.1016/j.carbpol.2023.121504. ISSN 0144-8617. PMID 37985092. S2CID 264314560.
- ^ Moghadas, Babak; Solouk, Atefeh; Sadeghi, Davoud (24 August 2020). "Development of chitosan membrane using non-toxic crosslinkers for potential wound dressing applications". Polymer Bulletin. 78 (9): 4919–4929. doi:10.1007/s00289-020-03352-8. ISSN 1436-2449. S2CID 221283821.
- Islam, S.; Bhuiyan, M.R.; Islam, M. Chitin and chitosan: Structure, properties and applications in biomedical engineering. J. Polym. Environ. 2017, 25, 854–866.
- Aranaz, I.; Harris, R.; Navarro-García, F.; Heras, A.; Acosta, N. Chitosan based films as supports for dual antimicrobial release. Carbohydr. Polym. 2016, 146, 402–410.
- ^ Aranaz, I.; Mengíbar, M.; Harris, R.; Miralles, B.; Acosta, N.; Calderón, L.; Sánchez, Á.; Heras, Á. Role of physicochemical properties of chitin and chitosan on their functionality. Curr. Chem. Biol. 2014, 8, 27–42.
- Vllasaliu, D.; Casettari, L.; Fowler, R.; Exposito-Harris, R.; Garnett, M.; Illum, L.; Stolnik, S. Absorption-promoting effects of chitosan in airway and intestinal cell lines: A comparative study. Int. J. Pharm. 2012, 430, 151–160.
- Rosenthal, R.; Günzel, D.; Finger, C.; Krug, S.M.; Richter, J.F.; Schulzke, J.-D.; Fromm, M.; Amasheh, S. The effect of chitosan on transcellular and paracellular mechanisms in the intestinal epithelial barrier. Biomaterials 2012, 33, 2791–2800.
- Hadwiger, Lee A. (2013). "Multiple effects of chitosan on plant systems: Solid science or hype". Plant Science. 208: 42–9. doi:10.1016/j.plantsci.2013.03.007. PMID 23683928.
- "Chitosan; Poly-D-glucosamine (128930) Fact Sheet" (PDF). Environmental Protection Agency (EPA). February 2001.
- Linden, James C.; Stoner, Richard J.; Knutson, Kenneth W.; Gardner-Hughes, Cecilie A. (2000). "Organic disease control elicitors". Agro Food Industry Hi-Tech. 11 (5): 32–4.
- "USDA NOP and EPA Rule on Chitosan, Federal Register/Vol. 72, No. 236/Monday, December 10, 2007/Rules and Regulation" (PDF). Archived from the original (PDF) on 11 December 2008.
- "Chitin and Chitosan Final Registration Review Decision, Document ID: EPA-HQ-OPP-2007-0566-0019". Regulations.gov. 11 December 2008. pp. 10–15.
- "Expert group for technical advice on organic production. Final report on plant protection (III)" (PDF). ec.europa.eu. European Commission. 18 November 2021. Archived (PDF) from the original on 25 July 2021.
- "Basic substances". ahdb.org.uk. Agriculture and Horticulture Development Board. Retrieved 18 November 2021.
- Goosen, Mattheus F. A. (1 June 1996). Applications of Chitan and Chitosan. CRC Press. pp. 132–9. ISBN 978-1-56676-449-0.
- Linden, J.C.; Stoner, R.J. (2005). "Proprietary Elicitor Affects Seed Germination and Delays Fruit Senescence" (PDF). Journal of Food, Agriculture & Environment.
- Smiley R.; Cook R.J.; Pauliz T. (2002). "Seed Treatment for Sample Cereal Grains" (PDF). EM 8797: Oregon State University. Archived from the original (PDF) on 5 September 2006.
{{cite web}}
: CS1 maint: location (link) - ^ Linden, J. C.; Stoner, R. J. (2007). "Pre-harvest application of proprietary elicitor delays fruit senescence". Advances in Plant Ethylene Research. pp. 301–2. doi:10.1007/978-1-4020-6014-4_65. ISBN 978-1-4020-6013-7.
- Mason, Mary E.; Davis, John M. (1997). "Defense Response in Slash Pine: Chitosan Treatment Alters the Abundance of Specific mRNAs". Molecular Plant-Microbe Interactions. 10 (1): 135–7. doi:10.1094/MPMI.1997.10.1.135. PMID 9002276.
- Klepzig, Kier D.; Walkinshaw, Charles H. (2003). "Cellular response of loblolly pine to wound inoculation with bark beetle-associated fungi and chitosan". treesearch.fs.fed.us. 030. Res. Pap. SRS-30.asheville, Nc: US Department of Agriculture, Forest Service, Southern Research Station. 9P.
{{cite journal}}
: CS1 maint: location (link) - O'Toole, Erin (10 September 2009). "Solution for Pine Bark Beetles May Help Front Range Trees". Morning Edition. Greeley, Colorado: KUNC 91.5 FM, NPR. Archived from the original on 24 September 2009. Retrieved 25 October 2009.
- Croteau, R.; Gurkewitz, S.; Johnson, M. A.; Fisk, H. J. (1987). "Biochemistry of Oleoresinosis: Monoterpene and Diterpene Biosynthesis in Lodgepole Pine Saplings Infected with Ceratocystis clavigera or Treated with Carbohydrate Elicitors". Plant Physiology. 85 (4): 1123–8. doi:10.1104/pp.85.4.1123. PMC 1054405. PMID 16665815.
- "Treatment of Plants with Chitosan Salts, 1989, Patent WO/1989/007395". wipo.int. Archived from the original on 5 August 2012.
- Stoner, R. (2006). "Progressive Plant Growing Has Business Blooming, Environmental and Agricultural Resources". NASA.gov. pp. 68–71. Archived from the original on 21 December 2015. Retrieved 17 April 2009..
- Linden, James C.; Stoner, Richard J. (21 October 2008). "YEA! Elicitor Response Comparison to Chitin / Chitosan in Mung Bean and Adzuki Bean Germination Experiments" (PDF).
- "Chitin/Chitosan, Farnesol/Nerolidol and Nosema locustae Final Registration Review Decision". Federal Register Notice of Availability. 73 (248). 24 December 2008.
- "Chitosan Exemption from the Requirement of a Tolerance". epa.gov. US Environmental Protection Agency.
- "Control Strategies to reduce postharvest decay of fresh fruits and vegetables". USDA.gov. Archived from the original on 16 July 2012.
- "Chitosan; Poly-D-glucosamine (128930) Fact Sheet". epa.gov. US Environmental Protection Agency. 2 May 2006. Retrieved 10 July 2006.
- Klepzig, Kier D; Strom, Brian L (20 August 2010). "Effects of a commercial chitosan formulation on bark beetle (Coleoptera: Curculionidae) resistance parameters in loblolly pine" (PDF). Journal of Entomological Science. 46 (2) (published 1 April 2011): 124–134. doi:10.18474/0749-8004-46.2.124. S2CID 12241255. Retrieved 6 March 2021 – via USDA Forest Service Southern Research Station.
. . . application of a commercially-prepared chitosan formulation to loblolly pine produced inconsistent responses in tree parameters associated with bark beetle resistance, suggesting that exogenously applied chitosan preparations have limited utility for managing bark beetles.
- ^ Yong, S. K; Shrivastava, M; Srivastava, P; Kunhikrishnan, A; Bolan, N (2015). "Environmental Applications of Chitosan and Its Derivatives". Reviews of Environmental Contamination and Toxicology Volume 233. Vol. 233. pp. 1–43. doi:10.1007/978-3-319-10479-9_1. ISBN 978-3-319-10478-2. PMID 25367132.
- Alan Woodmansey (19 March 2002). "Chitosan Treatment of Sediment Laden Water – Washington State I-90 Issaquah Project". Federal Highway Administration. U.S. Department of Transportation. Retrieved 10 July 2006.
- Rayner, Terry. "Fining and Clarifying Agents". Archived from the original on 16 June 2006. Retrieved 18 July 2006.
- Chorniak J (October 2007). "A clearer understanding of fining agents". Wine Maker Magazine. Retrieved 24 May 2014.
- Quintela, S; Villarán, M. C.; López De Armentia, I; Elejalde, E (2012). "Ochratoxin a removal from red wine by several oenological fining agents: Bentonite, egg albumin, allergen-free adsorbents, chitin and chitosan". Food Additives & Contaminants: Part A. 29 (7): 1168–74. doi:10.1080/19440049.2012.682166. PMID 22545592. S2CID 8215176.
- Escudero-Abarca, Blanca I.; Escudero-Abarca, M. Guadalupe; Aguilar-Uscanga, Patricia M.; Hayward-Jones, Patricia; Mendoza, Mario; Ramírez, Leticia (2004). "Selective antimicrobial action of chitosan against spoilage yeasts in mixed culture fermentations". Journal of Industrial Microbiology and Biotechnology. 31 (1): 16–22. doi:10.1007/s10295-004-0112-2. PMID 14747932. S2CID 250412.
- Ullah, Faheem; Othman, Muhammad Bisyrul Hafi; Javed, Fatima; Ahmad, Zulkifli; Akil, Hazizan Md. (1 December 2015). "Classification, processing and application of hydrogels: A review". Materials Science and Engineering: C. 57: 414–433. doi:10.1016/j.msec.2015.07.053. ISSN 0928-4931. PMID 26354282.
- ^ Feng, Peipei; Luo, Yang; Ke, Chunhai; Qiu, Haofeng; Wang, Wei; Zhu, Yabin; Hou, Ruixia; Xu, Long; Wu, Songze (2021). "Chitosan-Based Functional Materials for Skin Wound Repair: Mechanisms and Applications". Frontiers in Bioengineering and Biotechnology. 9: 650598. doi:10.3389/fbioe.2021.650598. ISSN 2296-4185. PMC 7931995. PMID 33681176.
- Baldrick, Paul (2010). "The safety of chitosan as a pharmaceutical excipient". Regulatory Toxicology and Pharmacology. 56 (3): 290–9. doi:10.1016/j.yrtph.2009.09.015. PMID 19788905.
- Guo, Baolin; Dong, Ruonan; Liang, Yongping; Li, Meng (1 November 2021). "Haemostatic materials for wound healing applications". Nature Reviews Chemistry. 5 (11): 773–791. doi:10.1038/s41570-021-00323-z. ISSN 2397-3358. PMID 37117664. S2CID 237551413.
- Ducheyne, Paul; Healy, Kevin; Hutmacher, Dietmar E.; Grainger, David W.; Kirkpatrick, C. James, eds. (2011). Comprehensive Biomaterials. Amsterdam: Elsevier. p. 229. ISBN 9780080552941.
- ^ Zhang, Yin-Juan; Gao, Bo; Liu, Xi-Wen (2015). "Topical and effective hemostatic medicines in the battlefield". Int J Clin Exp Med. 8 (1): 10–19. PMC 4358424. PMID 25784969.
- Singh R, Shitiz K, Singh A (December 2017). "Chitin and chitosan: biopolymers for wound management". International Wound Journal. 14 (6): 1276–1289. doi:10.1111/iwj.12797. PMC 7949833. PMID 28799228.
- ^ Rahmanian-Devin, Pouria; Baradaran Rahimi, Vafa; Askari, Vahid Reza (2021). "Thermosensitive Chitosan-β-Glycerophosphate Hydrogels as Targeted Drug Delivery Systems: An Overview on Preparation and Their Applications". Advances in Pharmacological and Pharmaceutical Sciences. 2021: 17. doi:10.1155/2021/6640893. PMC 8116164. PMID 34036263.
- Moghadas, Babak; Dashtimoghadam, Erfan; Mirzadeh, Hamid; Seidi, Farzad; Hasani-Sadrabadi, Mohammad Mahdi (19 January 2016). "Novel chitosan-based nanobiohybrid membranes for wound dressing applications". RSC Advances. 6 (10): 7701–7711. Bibcode:2016RSCAd...6.7701M. doi:10.1039/C5RA23875G. ISSN 2046-2069.
- Agnihotri, Sunil A.; Mallikarjuna, Nadagouda N.; Aminabhavi, Tejraj M. (2004). "Recent advances on chitosan-based micro- and nanoparticles in drug delivery". Journal of Controlled Release. 100 (1): 5–28. doi:10.1016/j.jconrel.2004.08.010. PMID 15491807.
- Aiedeh, K.M.; Taha, M.O.; Al-Khatib, H. (2005). "Evaluation of chitosan succinate and chitosan phthalate as enteric coating polymers for diclofenac sodium tablets". Journal of Drug Delivery Science and Technology. 15 (3): 207–211. doi:10.1016/S1773-2247(05)50033-9.
- Shukla, S. K; Mishra, A. K; Arotiba, O. A; Mamba, B. B (2013). "Chitosan-based nanomaterials: A state-of-the-art review". International Journal of Biological Macromolecules. 59: 46–58. doi:10.1016/j.ijbiomac.2013.04.043. PMID 23608103.
- Ryu, J. H; Hong, S; Lee, H (2015). "Bio-inspired adhesive catechol-conjugated chitosan for biomedical applications: A mini review". Acta Biomaterialia. 27: 101–15. doi:10.1016/j.actbio.2015.08.043. PMID 26318801.
- Federer, C; Kurpiers, M; Bernkop-Schnürch, A (2021). "Thiolated Chitosans: A Multi-talented Class of Polymers for Various Applications". Biomacromolecules. 22 (1): 24–56. doi:10.1021/acs.biomac.0c00663. PMC 7805012. PMID 32567846.
- ^ Fernandez J, Ingber D (February 2014). "Manufacturing of large-scale functional objects using biodegradable chitosan bioplastic". Macromolecular Materials and Engineering. 299 (8): 932–938. doi:10.1002/mame.201300426.
- Tampieri, A; Celotti, G; Landi, E; Sandri, M; Roveri, N; Falini, G (2003). "Biologically inspired synthesis of bone-like composite: Self-assembled collagen fibers/hydroxyapatite nanocrystals". Journal of Biomedical Materials Research. 67 (2): 618–25. doi:10.1002/jbm.a.10039. PMID 14566805.
- Tampieri, A; Celotti, G; Landi, E (2005). "From biomimetic apatites to biologically inspired composites". Analytical and Bioanalytical Chemistry. 381 (3): 568–76. doi:10.1007/s00216-004-2943-0. PMID 15696277. S2CID 25745619.
- Cheng, Q; Jiang, L; Tang, Z (2014). "Bioinspired layered materials with superior mechanical performance". Accounts of Chemical Research. 47 (4): 1256–66. doi:10.1021/ar400279t. PMID 24635413.
- Tajik, H; Moradi, M; Rohani, S. M.; Erfani, A. M.; Jalali, F. S. (2008). "Preparation of chitosan from brine shrimp (Artemia urmiana) cyst shells and effects of different chemical processing sequences on the physicochemical and functional properties of the product". Molecules. 13 (6): 1263–74. doi:10.3390/molecules13061263. PMC 6245338. PMID 18596653.
- Fernandez, J. G.; Ingber, D. E. (2012). "Unexpected strength and toughness in chitosan-fibroin laminates inspired by insect cuticle". Advanced Materials. 24 (4): 480–4. Bibcode:2012AdM....24..480F. doi:10.1002/adma.201104051. PMID 22162193. S2CID 205243157.
- Wyss Institute Communications (May 2014). "Promising solution to plastic pollution". Harvard Gazette, Harvard University, Boston, MA. Retrieved 23 May 2014.
- Shukla, S. K.; Mishra, A. K.; Arotiba, O. A.; Mamba, B. B. (2013). "Chitosan-based nanomaterials: A state-of-the-art review". International Journal of Biological Macromolecules. 59: 46–58. doi:10.1016/j.ijbiomac.2013.04.043. PMID 23608103.
- Lee, J. Y.; Choi, B.; Wu, B.; Lee, M. (2013). "Customized biomimetic scaffolds created by indirect three-dimensional printing for tissue engineering". Biofabrication. 5 (4): 045003. Bibcode:2013BioFa...5d5003L. doi:10.1088/1758-5082/5/4/045003. PMC 3852984. PMID 24060622.
- Selko A (6 March 2014). "Manufacturing 3-D objects just got easier with new bioplastic". Industry Week. Retrieved 24 May 2014.
- Fernandez, J. G.; Ingber, D. E. (2014). "Manufacturing of Large-Scale Functional Objects Using Biodegradable Chitosan Bioplastic". Macromolecular Materials and Engineering. 299 (8): 932–938. doi:10.1002/mame.201300426.
- "Manufacturing a solution to planet-clogging plastics". Hansjorg Wyss Institute for Biologically Inspired Engineering, Harvard University. 3 March 2014. Retrieved 5 June 2014.
- Zhao, Y; Xie, Z; Gu, H; Zhu, C; Gu, Z (2012). "Bio-inspired variable structural color materials". Chemical Society Reviews. 41 (8): 3297–317. doi:10.1039/c2cs15267c. PMID 22302077.
- "Chitosan bioplastic". Wyss Institute Communications, Hansjorg Wyss Institute for Biologically Inspired Engineering, Harvard University. 2014. Archived from the original on 24 May 2014. Retrieved 24 May 2014.
- Croisier, Florence; Jérôme, Christine (1 April 2013). "Chitosan-based biomaterials for tissue engineering". European Polymer Journal. Biobased Polymers and Related Materials. 49 (4): 780–792. doi:10.1016/j.eurpolymj.2012.12.009. ISSN 0014-3057.
- Kim, In-Yong; Seo, Seog-Jin; Moon, Hyun-Seuk; Yoo, Mi-Kyong; Park, In-Young; Kim, Bom-Chol; Cho, Chong-Su (1 January 2008). "Chitosan and its derivatives for tissue engineering applications". Biotechnology Advances. 26 (1): 1–21. doi:10.1016/j.biotechadv.2007.07.009. ISSN 0734-9750. PMID 17884325.
- Allison Sarubin-Fragakis, Cynthia Thomson (2007). The Health Professional's Guide to Popular Dietary Supplements. Academy of Nutrition and Dietetics. p. 96. ISBN 9780880913638.
- ^ Ríos-Hoyo, A; Gutiérrez-Salmeán, G (June 2016). "New Dietary Supplements for Obesity: What We Currently Know". Current Obesity Reports. 5 (2): 262–70. doi:10.1007/s13679-016-0214-y. PMID 27053066. S2CID 12071766.
- "Chitosan". Drugs.com. 12 February 2018. Retrieved 4 November 2018.
- Jull, Andrew B; Ni Mhurchu, Cliona; Bennett, Derrick A; Dunshea-Mooij, Christel AE; Rodgers, Anthony (2008). Jull, Andrew B (ed.). "Chitosan for overweight or obesity". Cochrane Database of Systematic Reviews (3): CD003892. doi:10.1002/14651858.CD003892.pub3. PMID 18646097.
- "Beware of Products Promising Miracle Weight Loss". US Food and Drug Administration. 5 January 2015. Retrieved 4 November 2018.
- ^ Al-Tayyar, Nasser A.; Youssef, Ahmed M.; Al-hindi, Rashad (2020). "Antimicrobial food packaging based on sustainable Bio-based materials for reducing foodborne pathogens: A review". Food Chemistry. 310: 125915. doi:10.1016/j.foodchem.2019.125915. ISSN 0308-8146. PMID 31841936.
- Poosapati, Aswani; Vadnala, Sudharshan; Negrete, Karla; Lan, Yucheng; Hutchison, John; Zupan, Mark; Madan, Deepa (15 April 2021). "Rechargeable Zinc-Electrolytic Manganese Dioxide (EMD) Battery with a Flexible Chitosan-Alkaline Electrolyte". ACS Applied Energy Materials. 4 (4). American Chemical Society (ACS): 4248–4258. doi:10.1021/acsaem.1c00675. hdl:11603/31329. ISSN 2574-0962. S2CID 234870771.
- Poosapati, Aswani; Negrete, Karla; Thorpe, Micah; Hutchison, John; Zupan, Mark; Lan, Yucheng; Madan, Deepa (6 April 2021). "Safe and flexible chitosan‐based polymer gel as an electrolyte for use in zinc‐alkaline based chemistries". Journal of Applied Polymer Science. 138 (33). Wiley: 50813. doi:10.1002/app.50813. hdl:11603/31330. ISSN 0021-8995. S2CID 233563261.
- Quaglia, Sofia (1 September 2022). "Crab and lobster shells could be used to make renewable batteries". the Guardian.
External links
- International research project Nano3Bio, focused on tailor-made biotechnological production of chitosans (funded by the European Union)
Types of carbohydrates | |||||||||||||||
---|---|---|---|---|---|---|---|---|---|---|---|---|---|---|---|
General | |||||||||||||||
Geometry | |||||||||||||||
Monosaccharides |
| ||||||||||||||
Multiple |
| ||||||||||||||